The "C" of PECM: Examining the Chemistry of Electrochemical Machining
- Kirk Abolafia
- Dec 16, 2024
- 4 min read
Disclaimer: This article assumes the reader has a fundamental understanding of electrochemical machining technology. We recommend preliminary reading for those unfamiliar with PECM's applications and methodology.
Interested in learning more? See our article in Stainless Steel World Magazine where we break down the chemistry of PECM using another example.
Under most circumstances, metal oxidization and corrosion on a part is seen as an undesirable outcome, as it can weaken the chemical bonds of a given metal, cause discoloration, a loss of material to the environment and, crucially, weakness in the material itself. As a case in point, manufacturers often produce materials, coatings, and part geometries specifically meant to deter or prevent corrosion for critical parts.
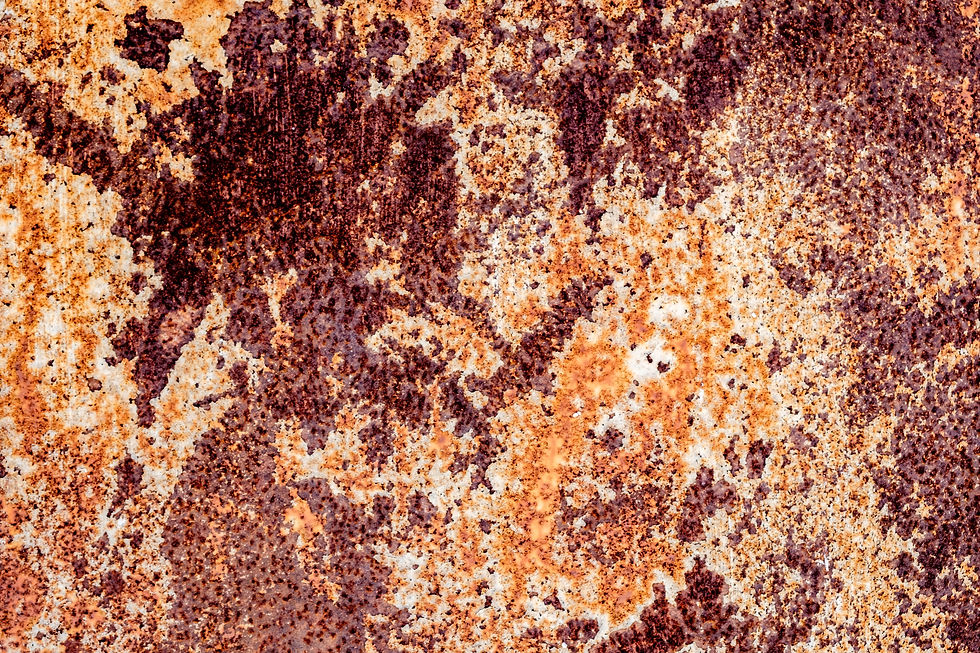
At its core, corrosion is the chemical process in which a refined metal becomes a more chemically stable oxide. The most common example of this chemical process is rust, in which iron oxidizes when exposed to water or air moisture for a prolonged period, developing a reddish-brown coloration.
Voxel’s pulsed electrochemical machining (PECM) technology is unique in that it takes advantage of the properties of corrosion to create unique manufacturing benefits not offered by conventional manufacturing processes, including non-thermal, non-contact material removal, tough material machining, high repeatability, and superfinished surfaces.
How, and why, does PECM utilize corrosion and electrolysis to machine components? This article will seek to answer those questions by providing an in-depth look at the chemical reactions that occur during the process, including oxidization and corrosion.
Disclaimer: This article assumes the reader has a fundamental understanding of electrochemical machining technology. We recommend reading other articles first, such as What is Electrochemical Machining.
During the PECM process, the anode surface is “machined” by first becoming oxidized— in which the atoms on the material’s surface lose one or more electrons, both changing its atomic structure, and separating it from the other atoms. The speed of this reaction is driven by the electrical current from the PECM power source which facilitates an imbalance of electrons between the positively charged anode (workpiece) and the negatively charged cathode (tool).
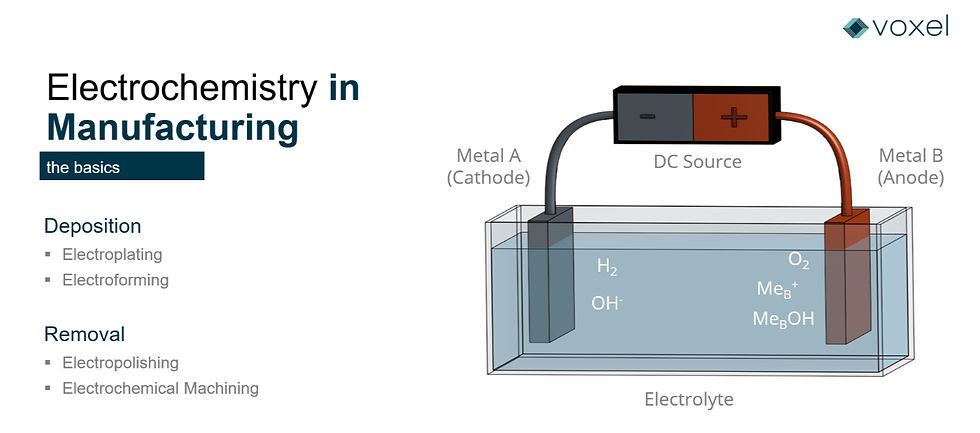
Despite the material removal process occurring at an atomic level, PECM can both machine and finish a part in a relatively short period of time, comparable with conventional machining processes.
This electrical charge causes the positively charged cations, as well as their electrons, to be removed from the anode surface and, under normal electrochemical circumstances, would be deposited on the negatively charged cathode surface.
In PECM, however, these cations are bonded with oxygen and hydrogen in the electrolytic fluid to form neutralized metal hydroxides before reaching their destination of the cathode. The lack of this deposit on the cathode is one of the key factors as to how PECM incurs minimal tool wear. These neutralized metal hydroxides are one of the waste products of the electrochemical reaction that are removed by the electrolytic fluid as it flows away from the workpiece area.
One additional reaction occurs—a reduction reaction, in which electrons form with hydrogen ions on the surface of the cathode that forms hydrogen gas, is another byproduct of ECM that is then removed from the process.
To best illustrate this reaction, let’s review a hypothetical equation in which Aluminum (Al) is machined via PECM, coming into contact with the oxygen molecules in water (H2O), losing its electrons to become Aluminum Oxide (Al2O3) alongside its spare electrons, some of which may become part of the hydrogen gas reaction on the surface of the cathode.

In this case, PECM will facilitate the aluminum to react with water in the electrolytic fluid and ultimately be broken up into two primary waste products: aluminum oxide and hydrogen gas. This results in an anodic and cathodic reaction, occurring in the workpiece and tooling, respectively.
In the anodic reaction (the actual material removal of the PECM process), aluminum molecules on the surface of the workpiece are broken off and separated into their cations and spare electrons:

The hydrolysis of Al+3 will produce aluminum oxide (Al2O3) alongside their protons and the spare electrons.
Here is an equation showing the aluminum cation and H2O ultimately forming aluminum oxide and the hydrogen cations:

However, this equation is not complete, as the anodic process mentioned beforehand must be coupled with a cathodic process at the cathode to fully balance the equation; the cationic form of hydrogen and the spare electrons will combine into hydrogen gas, the other byproduct of the electrochemical reaction.
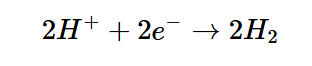
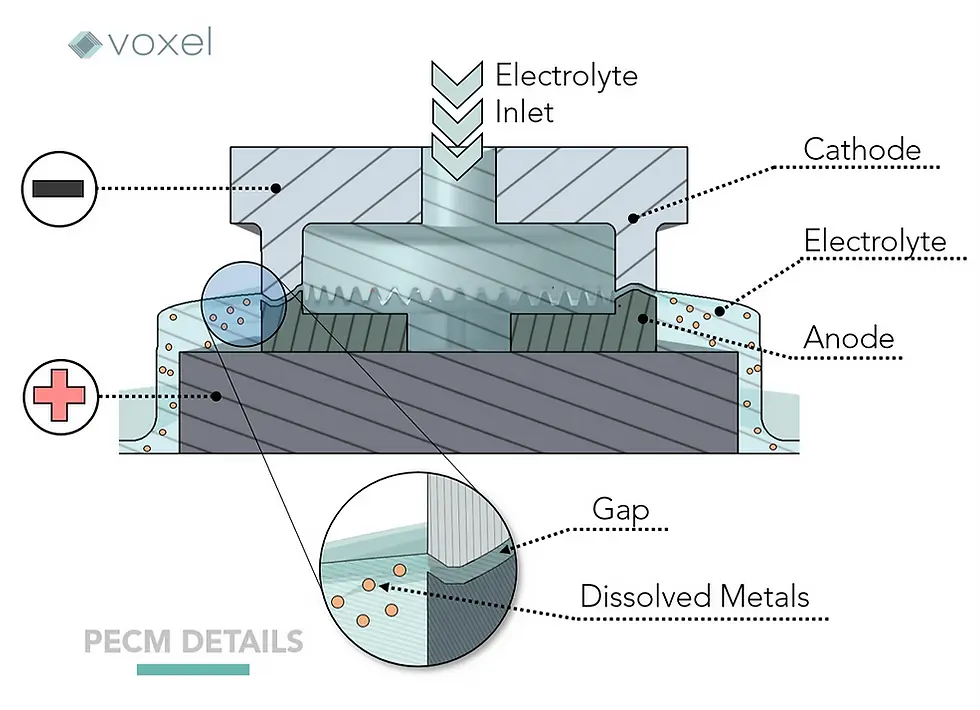
To summarize this reaction:
In the anodic reaction, aluminum on the surface of the anode (workpiece) is broken up by the charged electrolytic fluid into aluminum cations and its spare electrons.
In the cathodic reaction, hydrogen cations bond with the spare electrons to form hydrogen gas.
The end-result of the electrochemical reaction sees aluminum cations separated from the workpiece surface (the machining itself), and combine with electrons and water in the electrolytic fluid to form what are ultimately the waste products: neutralized aluminum oxide and hydrogen gas.

For additional examples, consider reading this article we produced for Stainless Steel Worlds where we share a similar equation of dissolving copper in the PECM process.
While the range of materials compatible with PECM go well beyond aluminum, the fundamentals of the PECM process stay largely unchanged, even for more complex materials. For example, PECM is capable of machining Inconel materials at a similar speed to aluminum or copper, which can be a combination of up to a dozen separate elements, including nickel, cobalt, iron, chromium and even refractory metals such as molybdenum. Machining advanced materials like Inconel still ultimately result in the materials losing electrons and combining with elements in the electrolytic fluid to produce neutralized metal hydroxides.
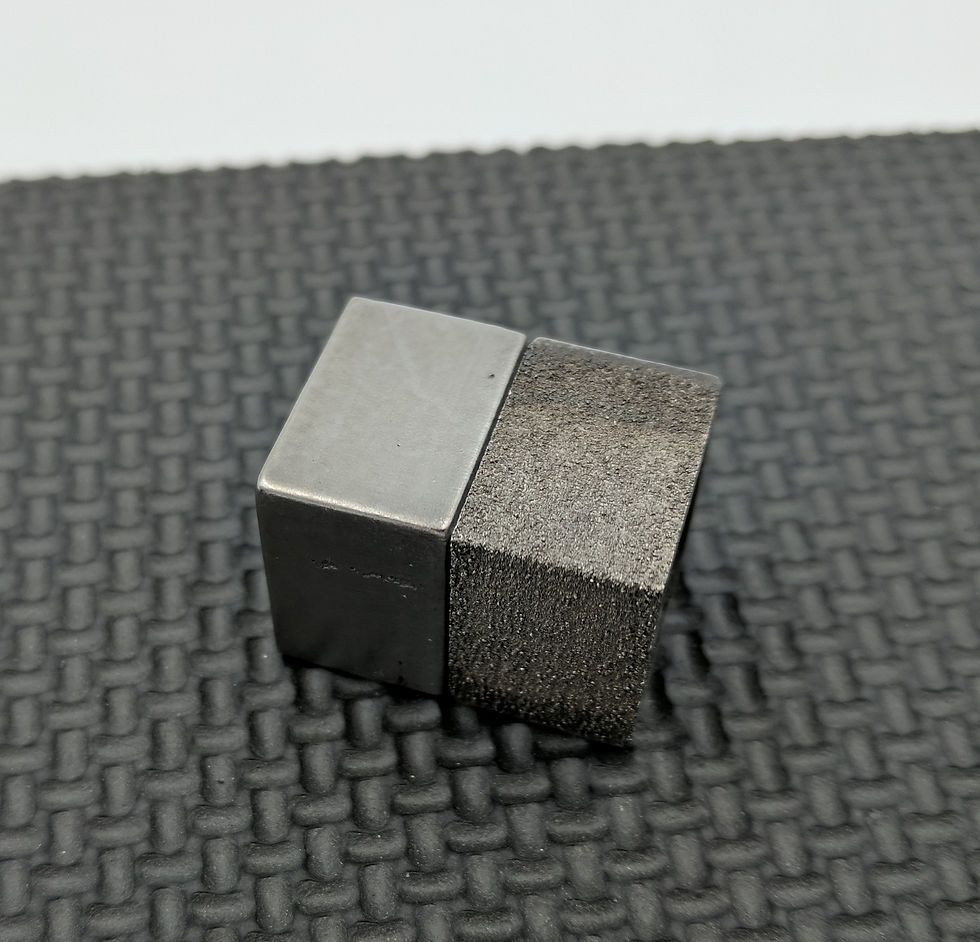
Under special circumstances, PECM can also dissolve workpiece materials that contain non-conductive elements, such as nano-structured metal matrix composites (MMCs). Although these abrasive, tough-to-machine materials may contain ceramics or polymers (materials that, in bulk, are non-conductive and therefore incompatible with PECM), the PECM process can still dissolve the metal material surrounding the composite particle, thereby removing the non-conductive particles alongside the metal molecules.
To learn more about PECM’s ability to machine advanced materials, consider reading this article.
Comments